The invention of PCR (Polymerase Chain Reaction) by chemist Kary Mullis at Cetus Corporation in the 1980s marked a revolutionary leap in biological research. Over three decades, PCR has evolved, adapting to new challenges and expanding its applications beyond laboratory confines. Its journey from classical PCR to real-time quantitative PCR (qPCR), and now to digital PCR (dPCR), reflects a technology that continually reinvents itself, maintaining its relevance in genetic identification, disease diagnosis, and a broadening range of fields.

Diverse Methods of PCR: From End-point to Digital
PCR’s diverse methodologies have their unique applications and significance. End-point PCR, the foundational method, offers simplicity but lacks quantitative capabilities. This method’s reliance on post-reaction techniques like gel or capillary electrophoresis makes it less efficient for quantitative analysis due to variable amplification efficiencies.
Advancements led to the development of qPCR and dPCR. qPCR utilizes intercalating dyes or fluorescent probes (e.g., TaqMan), enabling real-time DNA level monitoring during the PCR process. Commonly used dyes like SYBR® Green increase fluorescence intensity with each cycle, aiding in DNA quantification. Despite its popularity, SYBR® Green’s tendency for non-specific products and potential for false positives are notable drawbacks.
Digital PCR (dPCR) simplifies and refines the quantification process. By partitioning the sample into numerous PCR reactions, each with a single or no template molecule, dPCR provides a more precise count of the initial template molecules through positive and negative reaction assessments. This absolute quantification, a distinct advantage over the relative quantification of qPCR, eliminates the need for standard curves.
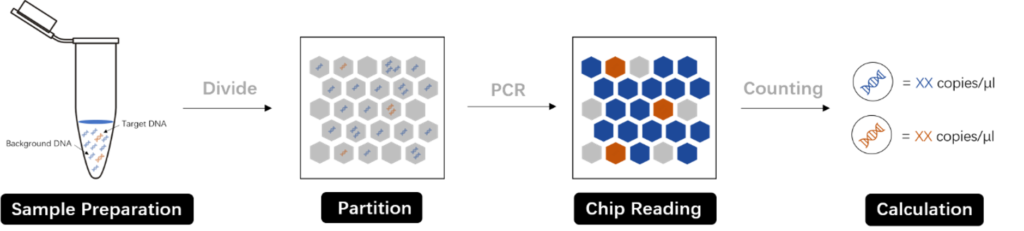
Comparing qPCR and dPCR: Precision and Applications
While both qPCR and dPCR quantify nucleic acids, they differ significantly in their approach and precision. qPCR, a more mature and widely available technology, is apt for high-throughput analysis and has a broader dynamic range. However, it excels mainly in relative quantification and is less effective in detecting minute concentration differences.
In contrast, dPCR’s strength lies in its accuracy, especially in detecting small variances in nucleic acid concentrations, essential in applications like gene copy number quantification in chromosomal rearrangements or monitoring circulating biomarkers in cancer patients. Furthermore, dPCR’s design makes it more resistant to inhibitors compared to qPCR.
Comparison | qPCR | dPCR |
---|---|---|
Principle | The ct value was determined according to the real-time fluorescence curve | The copy number of the target sequence was calculated statistically according to the signals of reaction units |
Quantitative type | indirect | direct |
Sensitivity | high | Extremely high |
Accuracy | Poor repeatability at low concentration | good repeatability |
Tolerance of inhibitory factors | Weak, greatly affected by amplification efficiency | Strong, less affected by inhibitory factors |
Application | gene expression level pathogen detection genotyping … | viral load CNV gene mutation rate … |
SYBR® Green is an intercalative DNA dye widely used in qPCR. As the PCR cycle continues, the fluorescence intensity of this dye increases, allowing one to quantify the DNA in the reaction. The SYBR Green method is lower in cost than the probe method and is more convenient to use. However, this method also has inherent drawbacks. The SYBR Green method produces more non-specific products, which can lead to high background and false positives. Nonetheless, this time-tested technique remains popular, and many researchers are loyal fans of the SYBR Green method and are willing to accept its minor flaws.
Although both qPCR and dPCR are capable of quantifying nucleic acids in a sample, they have an important difference. qPCR can only achieve relative quantification (for example, sample A has twice as many target sequences as sample B) unless a standard is used to generate a standard curve. Digital PCR itself is absolutely quantitative and does not require a standard curve. On the other hand, qPCR technology is more mature and well-known, and there are a large number of commercial products on the market to choose from. dPCR is still a relatively new technology at present. It is not as widely used as qPCR and is more expensive. Compared with dPCR, qPCR is more suitable for high-throughput analysis and has a wide dynamic range.
dPCR is generally more accurate. qPCR can easily distinguish two-fold concentration differences (such as 5 copies and 10 copies), but is less effective in the face of small differences. dPCR can theoretically identify copy numbers with a difference of less than 20%, such as 5 copies and 6 copies. This precision is particularly helpful in areas such as quantifying copy numbers of genes involved in chromosomal rearrangements, or quantifying circulating biological markers in the blood of cancer patients. Because dPCR dispenses the equivalent of diluting the sample and reads data at the end of the reaction, dPCR is more resistant to inhibitors than qPCR.
Conclusion: The Ever-Evolving PCR Landscape
The PCR landscape is a testament to continuous innovation in molecular biology. Each method, from end-point PCR to the precise dPCR, offers unique benefits, addressing specific needs in research and diagnostics. As technology advances, the potential applications of PCR in various fields continue to grow, solidifying its position as a cornerstone in molecular biology.